Share This Article
Today, cell cultures are considered essential tools in biomedical research and regenerative medicine. They allow us to create disease models or biological systems for drug screening more quickly and economically than traditional animal models. However, it has taken more than a century for scientists to fine-tune cell culture techniques in order to obtain physiologically relevant systems.
After many hours of work and dedication, researchers have been able to upgrade from the most rudimentary cultures, where cells simply floated in saline media, to sophisticated artificial tissues. Scientists are now able to grow, and even bio-print, different cell types in a three-dimensional scaffold, mimicking some fundamental aspects of the different organs of the body.
The development of complex culture systems has been possible, in part, thanks to the more exhaustive knowledge we have of the extracellular matrix, a protein-based scaffold that not only provides physical support to the cells but also a substrate for cell signaling and cell-to-cell interactions.
Adedamola (Dammy) Olayanju is a doctor of Pharmacology and Translational Medicine who works as a scientist and technical expert at Manchester BIOGEL, an award-winning UK start-up and a leader in the design and production of synthetic extracellular matrices.
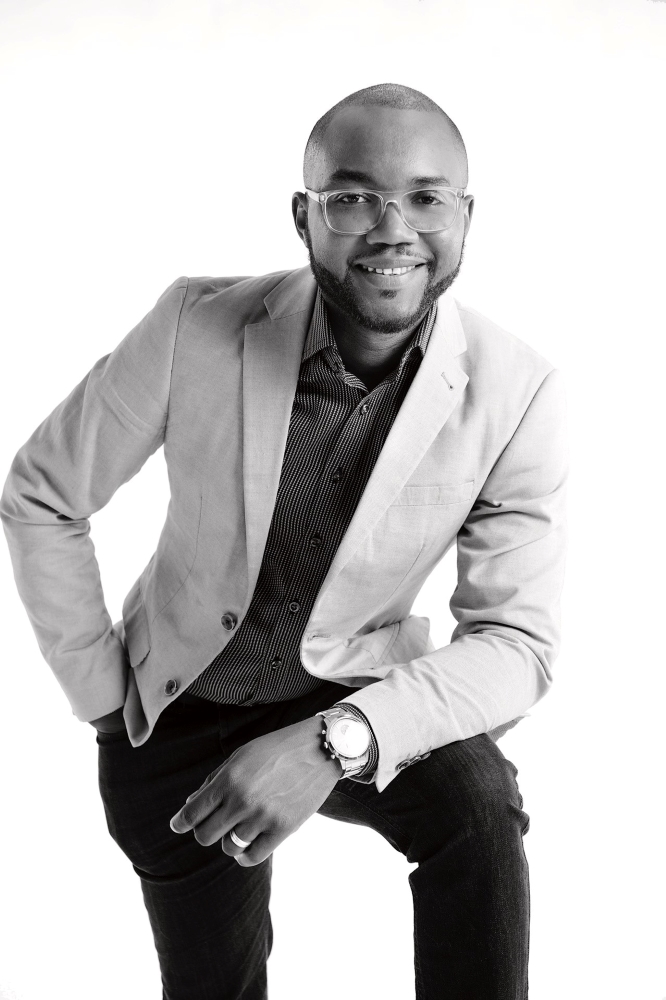
SciGlam: How do cell cultures and tissue engineering contribute to biomedical research and human health?
Dammy Olayanju: Cell cultures are a key aspect of biomedical sciences. They are fundamental in many processes, from vaccine development (as we have seen with the recent COVID outbreak) where we can grow antibodies in cells and then multiply them; up until drug screening because a lot of new drugs need to be discovered and validated in cultures before moving into traditional animal research, and then into clinical applications. Apart from drug related applications, the role of cell cultures is also vital in cell therapy and regenerative medicine. In fact, big research, most times, starts with cell cultures.
Big research, most times, starts with cell cultures.
What prompted scientists to generate artificial tissues?
Every organ in the body is composed of different tissues, and each of these tissues is composed of different cell types that work together to provide the function of the organ. Traditionally, we have tried to study this behaviour in laboratories with cultures of isolated cells, where we just needed some plastic dishes to contain the cells and the medium with nutrients to maintain them. But the issue with this is the lack of a proper environment. To be able to culture the cells in a dish, scientists would have to destroy the environment that they had in the original tissue.
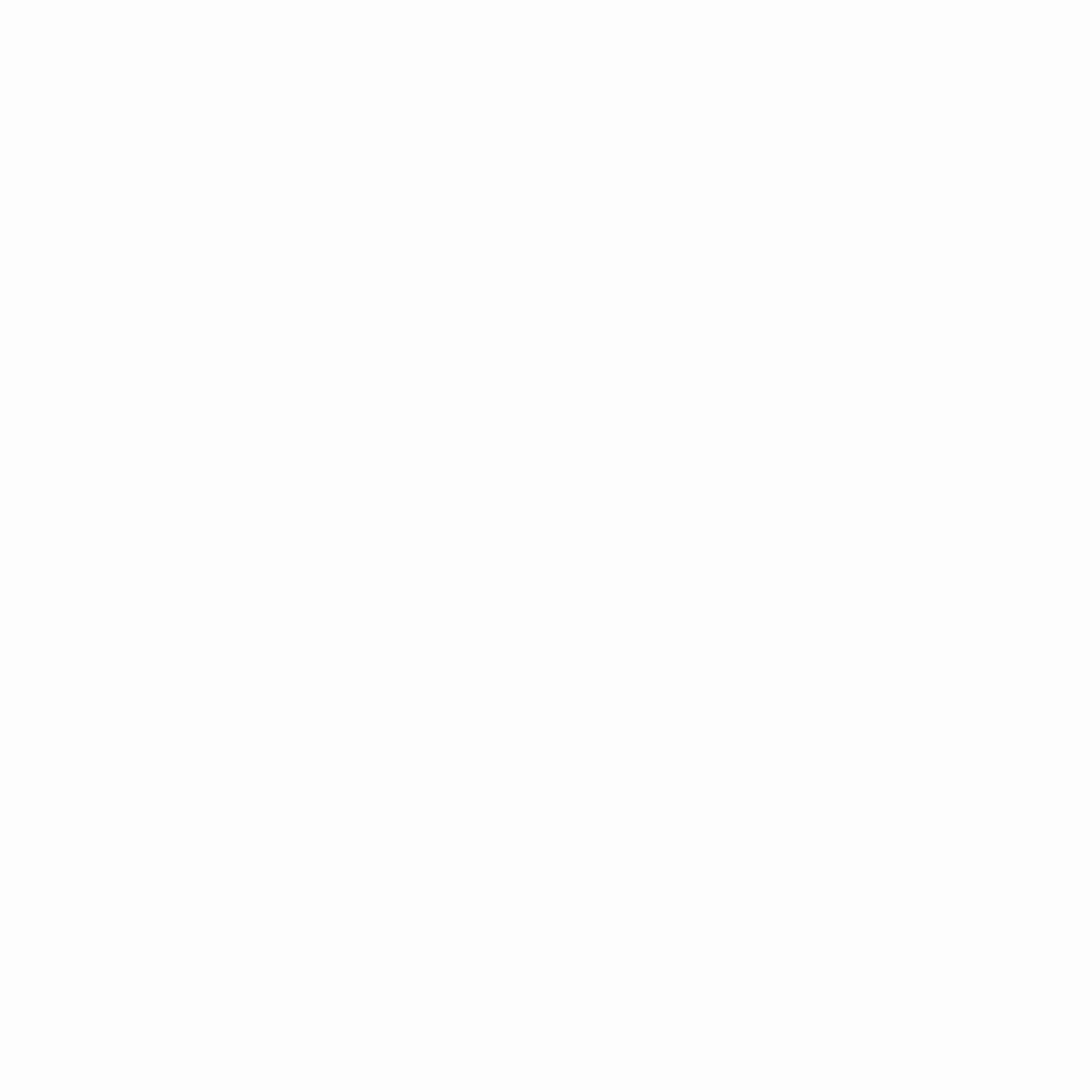
Cells need to be in permanent communication with each other and with the environment they belong to in order to achieve the maximal response and behaviour—and maximize their chances of staying alive. This native environment is what we call the extracellular matrix. Basically, if you are used to living in Montréal and you come to live in England tomorrow, you will need some time to re-establish yourself in your new environment, and the more accommodating this environment is, the easier it will be for you to function again.
For this reason, in the past few years there has been an advancement in technology that comes up with different extracellular matrices to try to replicate this native environment. Like very soft hydrogels that serve as scaffolds, or mechanical support, for cells to grow in. But mechanical support is not all they need, and the latest research has demonstrated that the structural and chemical features of these matrices are critical for the cells. So, now, we are entering a new generation where we create more organotypic cultures that can really mimic the cells’ native environment. We can also use 3D bio-printers to create three-dimensional structures with these synthetic gels and add different cell types (bio-inks) to the different layers of the structure, providing the cells in culture with a more appropriate environment.
Extracellular matrix, bio-printing, bio-ink… It all sounds like science fiction, doesn’t it?
As scientists, we often talk about “the future of science,” but I believe the future of science is here, now.
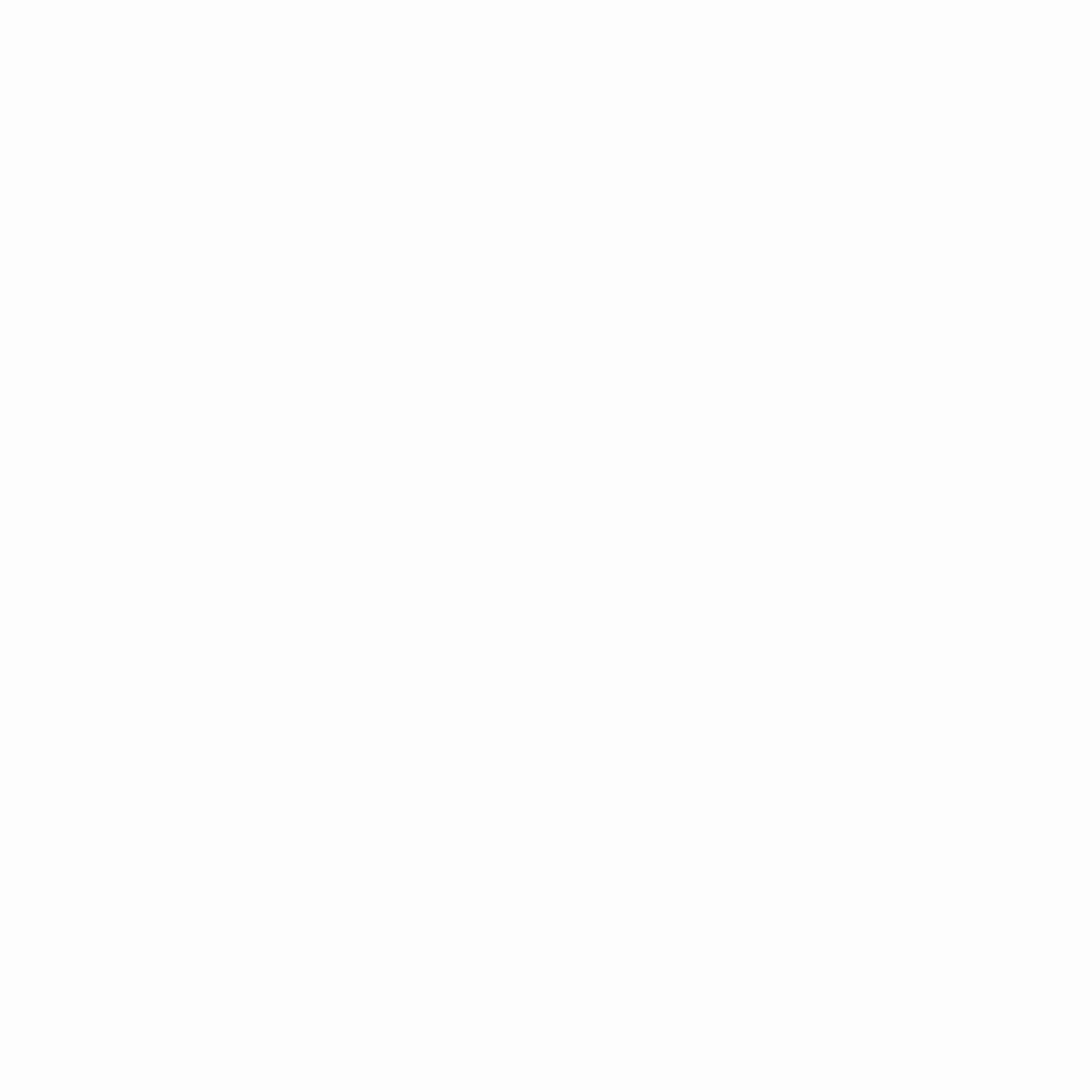
What type of therapies can benefit from extracellular matrix research?
Extracellular matrix research fits into a lot of different pathologies. In most illnesses, cells are growing in pathological environments. For example, in cancer formation (oncogenesis) the extracellular matrix plays a key role in mechanotransduction pathways. Changing the mechanical and chemical conditions, like pH and oxygenation levels, can replicate the tumor microenvironment and improve our understanding of the pathology; if we use an extracellular matrix that is stiffer, we mimic the environment of cancer; if we use a softer matrix, we mimic healthy conditions.
The environment is very important in cardiac related pathologies as well, where some therapeutic strategies use stem cells that have to be grown and differentiated to tissue-specific cells in an extracellular matrix before being injected into the patient.
As scientists, we often talk about “the future of science,” but I believe the future of science is here, now.
Why have the biogels you produce become so popular within the field?
Many research labs use animal-derived matrices to grow their cells; these extracellular matrices, although useful in pre-clinical research, come with a lot of limitations. Regarding pre-clinical research, they are not optimal because they have a lot of batch-to-batch variability, and so it leads to a lack of reproducibility between experiments. Moreover, the source of these matrices is also a limitation, they have a tumorigenic source—these matrices are extracted from tumors induced in the animals—and for this reason may not represent healthy environments. But most importantly, they do not have translational relevance, this means that they cannot be used in the clinic in terms of cell therapy because these components have an animal source. The genetics of animals are not the same as humans.
To be able to really represent an environment, you need extracellular matrices that you can fine-tune in terms of mechanical stiffness to mimic the tissue you want to study. For example, bone stiffness is different from that of brain and liver, or kidney tissue. You also want matrices that you can modify according to the pathology you want to study—to be able to mimic the pathology you want to study in a dish as much as possible—this is not possible with animal-derived matrices because, yes, they provide some mechanical support for cells to grow in a three-dimensional manner, but you basically don’t know what’s in it. Contrarily, synthetic matrices can be customized: mechanical strength, growth factors; we know the full composition. On top of that they are clinically translatable.
Peptide-based hydrogel shows biocompatibility with many different cell types, and you can fine-tune it both mechanically and chemically. You can define the growth factors and make them very specific.
On the other hand, they come ready to use. You can use them at room temperature; they do not need to be shipped frozen, and don’t need to be diluted. It makes things easier for scientists. They are extremely busy people, so any help is very much appreciated (laughs).
You are Dr. Dammy Olayanju by day and Dr. Dammy Pilgrims by night. How is it to have a double identity?
Music to me is a way of having a good work-life balance. I grew up playing few musical instruments and with the passion to help other musicians grow.
In 2016, I founded African Piano Lessons to teach musicians the art of African music techniques. I share some classes on our YouTube channel, which now has over 18000 subscribers.
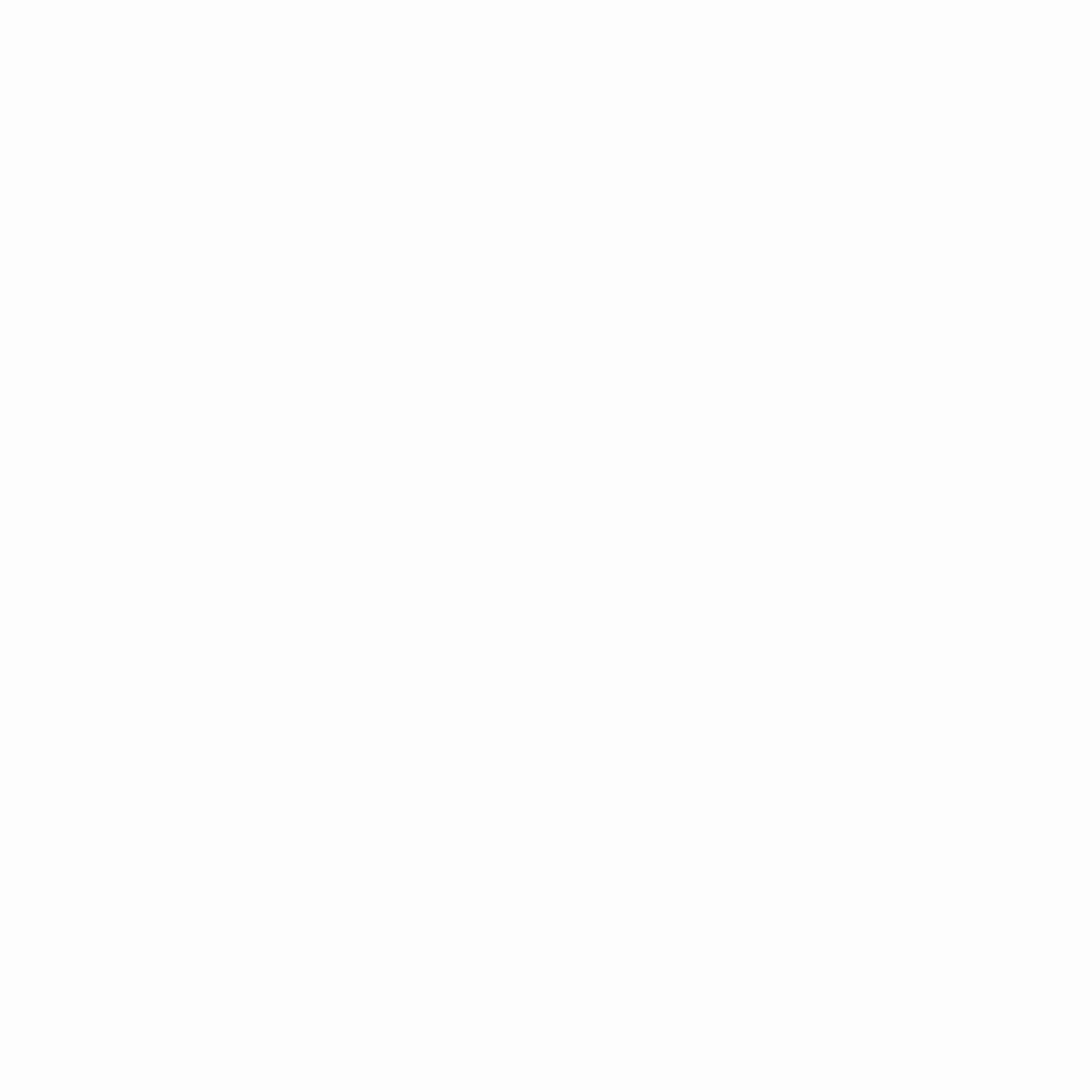
I also sing and play in an African band, Dr Dammy and the Pilgrims. We mostly do a fusion of African and contemporary gospel music. We usually play in the UK and, occasionally, at international events.
I like to spend my time off playing with the band. Music is very relaxing to me.